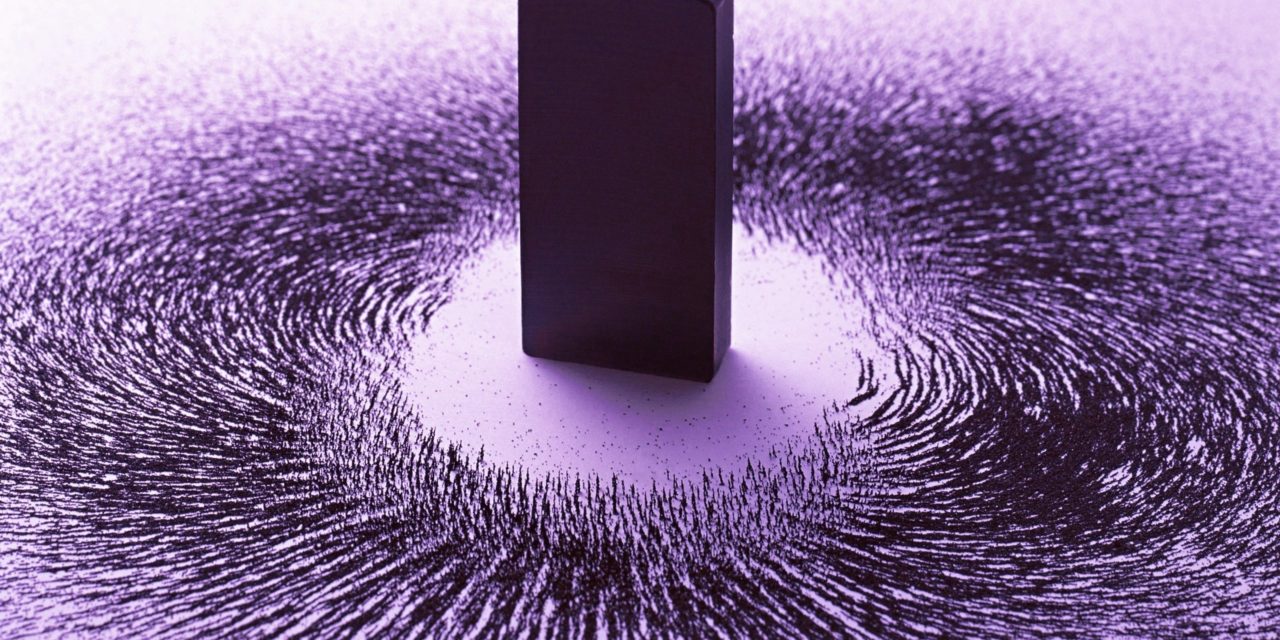
Electromagnetism is the study of electric and magnetic fields and their interactions with matter. The course explores the fundamental principles described by Maxwell's equations, which unify electricity and magnetism into a single theoretical framework. Topics typically include electrostatics, magnetostatics, electric and magnetic fields in matter, electromagnetic induction, and electromagnetic waves. Students learn about concepts such as Gauss’s law, Ampère’s law, Faraday’s law, and the Lorentz force. The course also covers boundary conditions, potentials, energy conservation, and the behavior of fields in conductors, insulators, and waveguides. Overall, electromagnetism provides a foundational understanding of many physical phenomena and forms the basis for technologies ranging from motors and generators to wireless communication.
Objectives:
- To provide a deep understanding of the fundamental laws governing electric and magnetic fields.
- To develop the ability to apply Maxwell’s equations to physical systems.
- To analyze how electric and magnetic fields interact with materials and charges.
- To explore the generation, propagation, and properties of electromagnetic waves.
- To build a foundation for applications in physics, engineering, and modern technology.
Learning Outcomes:
- State and explain the physical meaning of Maxwell’s equations in both differential and integral forms.
- Analyze electrostatic and magnetostatics fields using vector calculus and symmetry arguments.
- Solve problems involving electric and magnetic fields in vacuum and in various media.
- Apply boundary conditions to determine field behavior at interfaces.
- Understand the principles of electromagnetic induction and the operation of devices like transformers and generators.
- Describe the propagation of electromagnetic waves in different media and understand their energy and momentum transport.
- Use the concepts of electric potential, magnetic vector potential, and electromagnetic energy density effectively in problem solving.
Academic Year 2024-2025
Lecturer: Alfred MUKURU
- Teacher: content creator
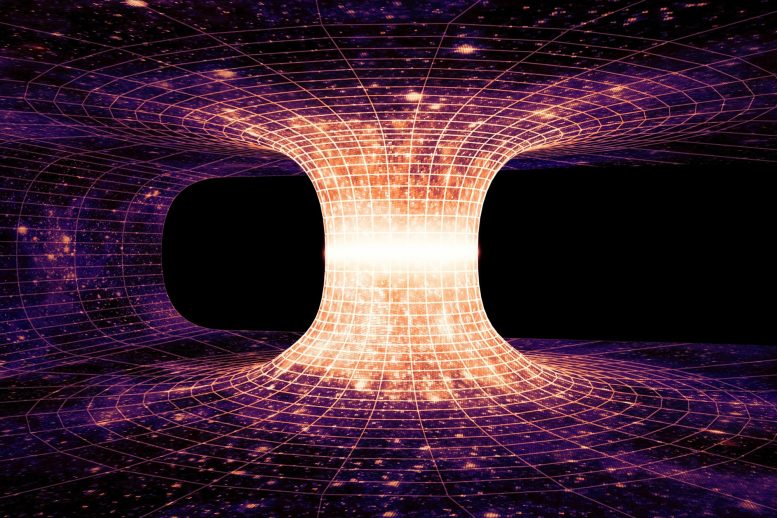
Statistical physics bridges microscopic particle behavior with macroscopic physical properties using probabilistic methods. It explains how systems with a vast number of particles, such as gases or solids, exhibit predictable thermodynamic behavior despite the randomness at the microscopic level. The course typically covers key concepts like the microcanonical, canonical, and grand canonical ensembles, the laws of thermodynamics, entropy, partition functions, and fluctuations. It also explores applications to ideal and real gases, phase transitions, quantum statistics (Bose-Einstein and Fermi-Dirac), and critical phenomena. Overall, statistical physics provides a fundamental framework for understanding matter in equilibrium from a microscopic point of view.
Objectives:
- To develop an understanding of how macroscopic physical properties emerge from microscopic particle interactions.
- To introduce the formalism of statistical ensembles and their application to physical systems.
- To apply probability theory and thermodynamic principles to analyze systems in equilibrium.
- To explore the statistical foundations of thermodynamic laws.
- To examine classical and quantum distributions and their implications for physical behavior.
Learning Outcomes:
- Explain the principles and assumptions underlying statistical mechanics.
- Derive and interpret key thermodynamic quantities using statistical methods.
- Distinguish between different statistical ensembles and apply them to relevant problems.
- Analyze systems of particles using classical and quantum statistical distributions (Maxwell-Boltzmann, Bose-Einstein, Fermi-Dirac).
- Apply statistical mechanics to real-world systems such as gases, magnets, and phase transitions.
- Solve problems involving entropy, energy distributions, and fluctuations in equilibrium systems.
Academic Year 2024-2025
Lecturer: Augustin SIWEGUSA
- Teacher: content creator
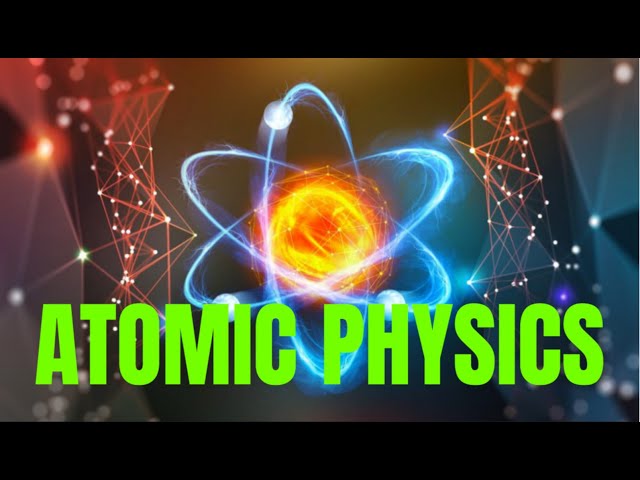
The Atomic Physics course explores the structure, behavior, and interactions of atoms, focusing on the principles that govern atomic systems and their energy levels. Topics include the historical development of atomic models, quantum theory, electron configurations, atomic spectra, and fine structure. The course examines the interaction of atoms with electric and magnetic fields (Zeeman and Stark effects), spin-orbit coupling, and the principles of atomic transitions and radiation. It provides a foundation for understanding atomic-scale phenomena and their applications in spectroscopy, lasers, quantum optics, and modern technology. Emphasis is placed on both theoretical understanding and experimental techniques used in atomic physics.
Objectives
To understand the fundamental structure and properties of atoms based on quantum mechanics.
-
To trace the historical development of atomic models from classical to modern quantum theory.
-
To analyze atomic spectra and understand the origin of spectral lines.
-
To study the effects of external fields on atomic energy levels (e.g., Zeeman and Stark effects).
-
To introduce experimental techniques and applications related to atomic transitions and interactions.
Explain the structure of atoms using quantum mechanical principles.
-
Describe and compare atomic models such as Bohr’s model and the quantum mechanical model.
-
Interpret atomic spectra and calculate energy level transitions.
-
Analyze the effects of magnetic and electric fields on atomic systems (Zeeman and Stark effects).
-
Understand the concepts of spin, fine structure, and spin-orbit coupling.
-
Apply atomic physics concepts to technologies such as lasers, atomic clocks, and spectroscopy.
-
Solve problems involving electron configurations, selection rules, and transition probabilities.
Academic Year 2024-2025
Lecturer: Augustin SIWEGUSA
- Teacher: content creator
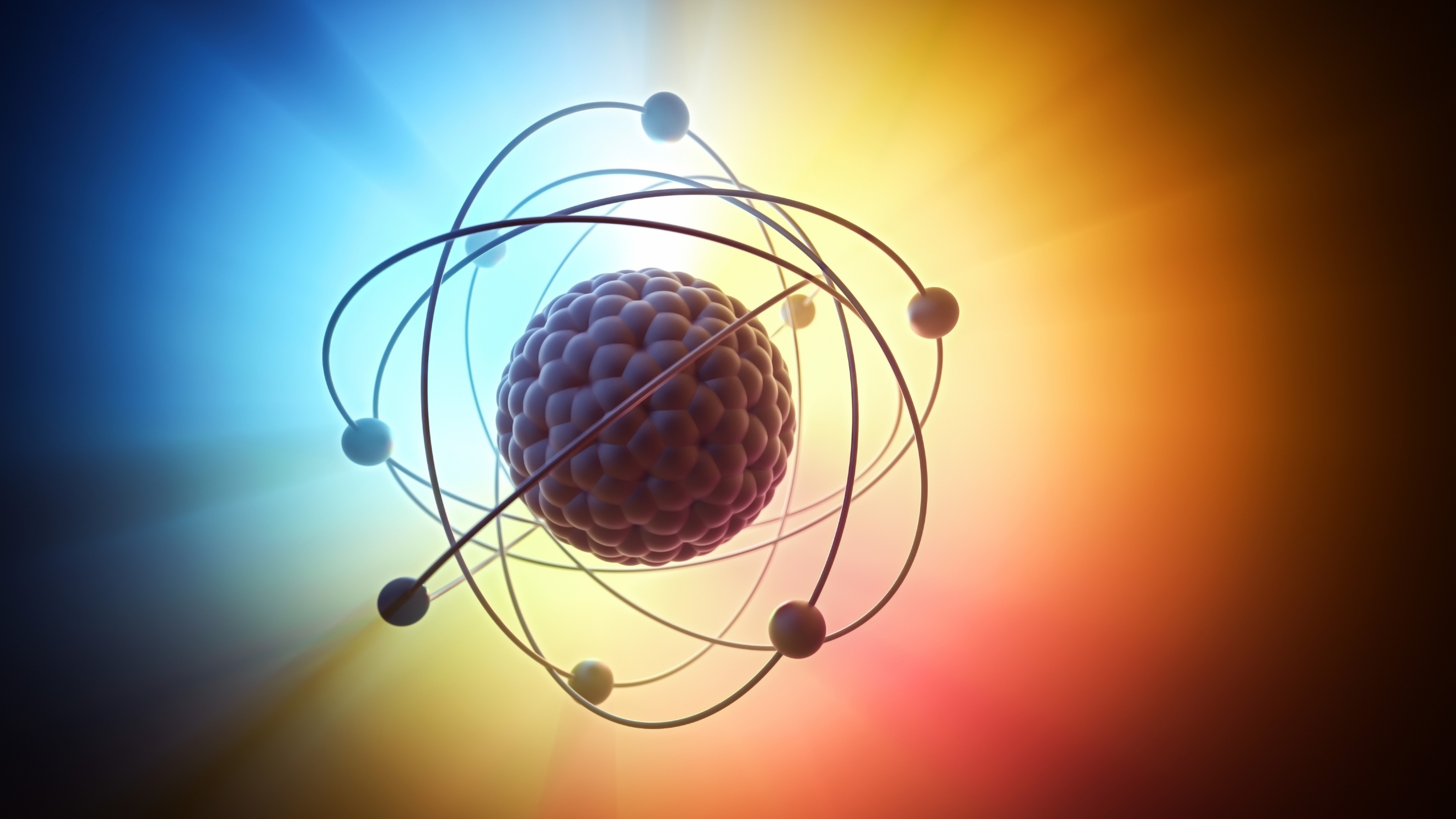
The Quantum Mechanics course provides a foundational understanding of the principles and mathematical framework that govern the behavior of matter and energy at atomic and subatomic scales. It explores the departure from classical physics, introducing core concepts such as wave-particle duality, quantum states, uncertainty principle, superposition, and quantization. The course covers the Schrödinger equation, quantum operators, probability densities, and applications to systems like the hydrogen atom, potential wells, and harmonic oscillators. Emphasis is placed on the theoretical development of quantum theory and its implications for modern physics, chemistry, and emerging technologies like quantum computing.
Objectives:
- To introduce the fundamental principles and postulates of quantum mechanics.
- To understand the mathematical formalism used to describe quantum systems.
- To explore the physical interpretation of wavefunctions, operators, and observables.
- To apply quantum mechanics to solve problems involving simple systems such as particles in potential wells and atoms.
- To build a conceptual foundation for advanced study and applications in modern physics, chemistry, and quantum technologies.
- Explain key concepts in quantum mechanics including wave-particle duality, superposition, and uncertainty.
- Formulate and solve the time-dependent and time-independent Schrödinger equations for basic quantum systems.
- Interpret the physical meaning of wavefunctions, probability densities, and quantum operators.
- Apply quantum principles to analyze systems such as infinite/finite potential wells, the harmonic oscillator, and the hydrogen atom.
- Use mathematical tools such as linear algebra, differential equations, and complex numbers in quantum contexts.
- Understand and discuss the implications of quantum mechanics in modern scientific and technological developments.
Academic Year 2024-2025
Lecturer: Augustin SIWEGUSA
- Teacher: content creator
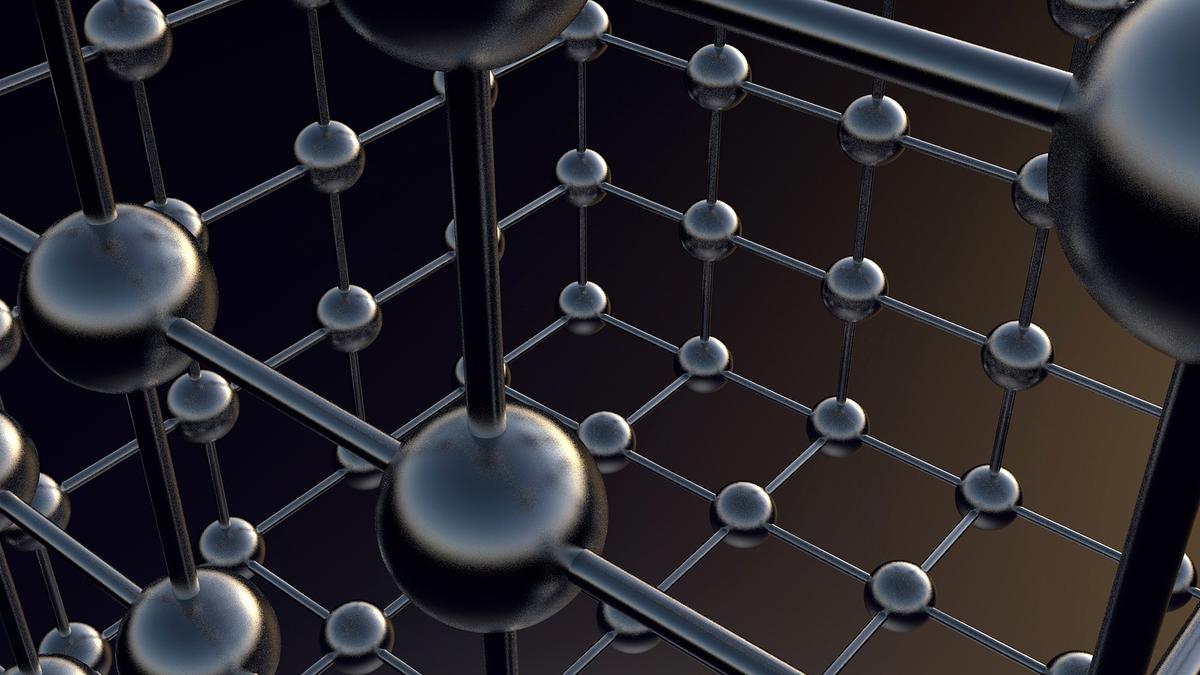
The Solid State Physics course focuses on the physical principles governing the behavior of solids, particularly crystalline materials. It explores the structural, electrical, thermal, magnetic, and optical properties of solids by applying quantum mechanics, statistical physics, and electromagnetism. Key topics include crystal lattice structures, band theory, semiconductors, superconductivity, and magnetism. The course provides a foundational understanding of how microscopic properties of atoms and electrons lead to macroscopic material behavior, with applications in modern technology such as electronics, nanotechnology, and materials science. Emphasis is placed on both theoretical frameworks and practical implications in research and industry.
Objectives:
- To introduce the fundamental concepts and theoretical models used to describe the properties of solid materials.
- To explore the structure and dynamics of crystal lattices and their influence on material behavior.
- To understand electronic, thermal, magnetic, and optical properties of solids.
- To analyze the behavior of conductors, semiconductors, and insulators using band theory.
- To develop problem-solving skills applicable to real-world materials and technologies in physics and engineering.
- Describe the structure of crystalline solids and determine lattice types and unit cells.
- Apply quantum and statistical mechanics to explain the properties of electrons in solids.
- Use band theory to differentiate between conductors, semiconductors, and insulators.
- Analyze thermal properties such as specific heat and thermal conductivity in solids.
- Explain magnetic and dielectric behavior in various materials.
- Solve problems related to solid-state phenomena and interpret experimental data in the context of theoretical models.
- Relate solid-state principles to practical applications in electronics, materials science, and nanotechnology.
Academic Year 2024-2025
Lecturer: Augustin SIWEGUSA
- Teacher: content creator